Theory
Earth's Atmosphere
CONTENT - The Earth's atmosphere. A tutorial in our series about the Earth |
|
Atmosphere is part with the magnetosphere of Earth shield against outer space. Earth's atmosphere reaches up to 390 000 miles away on Earth's nightside, or 50 times the diameter of our planet as that thin cloud of hydrogen atoms, or 'geocorona' encompasses our Moon. It's due to Sun interacting with hydrogen atoms through a particular wavelength of ultraviolet light called Lyman-alpha, which the atoms can both absorb and emit. Recent studies about greenhouse effect have shown that Earth's atmosphere provide a natural and beneficial greenhouse effect as it traps a part of Sun's heath radiating back to space. Earth's atmosphere is 78 percent nitrogen, 21 percent oxygen, and 1 percent other gases (mostly argon and trace elements of other gases). Earth's atmosphere have endured a three-step history, first being made of hydrogen, nitrogen, carbon dioxide, and methane. This primitive atmosphere might have been blown up by solar wind and a new atmosphere originated from the outgasing of Earth's crust. It was made of carbon dioxide, sulfur dioxide, and other gases. The present atmosphere is due to the apparition of the living organisms: they removed much of the carbon dioxide and produced oxygene. The Earth thermal budget is performed like rising thermal energy is absorbed by particles in the atmosphere, exciting them at the atomic level causing the atmospheric gases to vibrate in definite patterns determined by the bond between atoms. These vibrating bonds are where heat is absorbed and retained. Intense vibrating gas molecules then migrate toward outlets in the atmosphere, called 'infrared windows', where heat is released into space, helping our planet maintain a balanced temperature, with no gases exchanged. It looks like that when the solar radiation along a 11-year cycle decreases, the stratospheric ozone decreases too as the Earth's atmosphere thermal structure is variedly affected through the varied wavelengths of Sun's light. The lower atmosphere, on the other hand, has its temperature augmented through any increase in the solar input. Conditions in the stratosphere near the North Pole influence conditions in the mesosphere near the South Pole days or weeks later even going so far as to influence the transition between seasonal conditions as vertical motion of the air, and between hemispheres make that weather events, like thunderstroms, near Earth's surface trigger changes in the upper atmosphere. Gravity waves, or oscillations in the air usually caused by weather and winds near Earth’s surface, affect the entire circulation of the middle and upper atmosphere. Atmospheric gravity waves are caused by the convecting
and uplifting of air masses, such as when air is pushed up by mountain ranges and these waves play major roles in transferring energy from the lower atmosphere to
the mesosphere. Atmospheric gravity waves are generated by terrestrial weather and they impact the transport of energy and momentum from the lower atmosphere into near-Earth space, a fundamental question in heliophysics. Gravity or buoyancy waves are a physical phenomenon where waves are generated in any fluid including the atmosphere, and usually created by obstructions or disturbance to airflow such as mountain ranges or by opposition between air masses with different temperatures. On Earth, the oxygen we breathe is made of two atoms of oxygen (O2), but in space the sun’s rays break down (O2) into single oxygen atoms, creating atomic oxygen
You'll have to know too that the thickness of the Earth's atmosphere is varying function of the solar cycles. The Sun's activity varies along a 11-year cycle, with a maximum and then a minimum of activity. The Earth's atmosphere, at the time of a minimum is loosing up to 125 miles (200 km)! This height is then regained back to normal -and to its role of protecting the Earth- when the Sun's activity is back to increasing! When the atmosphere is loosing that part of its height, that means too a less drag exerted upon the artificial satellites in a low-Earth orbit, with the residual atmospheric friction working less on the satellites and not bringing it, like usual, to loose altitude and eventually deorbit by themselves! Another reaction of the Earth's atmosphere to the Sun radiation is that related to the absorption of the X-rays and UV. The upper atmosphere heats up and expands, leading too to the orbiting satellites to be affected. In other words, the ionosphere and thermosphere are heavily influenced by the energy carried through space by the solar wind, included during transient episodes like the solar flares which, for example, may increase by 50 percent the atmospheric drag during one day at a altitude of 300 miles (480km). Solar flares can cause a sharp increase in the number of ionized particles as gravity pulls the denser plasma of ionized gas down toward Earth to lower altitudes that are less dense, a unstable configuration of the atmosphere. During a solar energetic event, the thermosphere absorbs energy as infrared radiation from carbon dioxide (CO2) and nitric oxide (NO), the two most efficient coolants in the thermosphere, re-radiate 95 percent of that energy back into space. Under particularly tumultuous conditions, this energy can heat up the thermosphere, which then expands, exerting an atmospheric drag on orbiting spacecraft as the spacecraft prematurely lose altitude and plunge to Earth. With the ionosphere disrupted, which is where satellites are orbiting, it is the communications signals which are thrown off. In the middle and upper atmosphere, current circulation or waves are passing on the effects of solar events, generally and affecting the general weather patterns under
click to a diagram of Earth's atmosphere
Atmosphere has a layered structure. The thin Earth’s atmospheric limb known as the 'MLTI region,' for 'Mesosphere and Lower Thermosphere/Ionosphere,' at 10-110 miles of altitude, is home to the International Space Station and hundreds of satellites in the Low Earth Orbit. It is also where the solar energy mostly impacts Earth’s atmosphere. The lower thermosphere at between 124 and 236 miles of altitude, is where cosmic radiation can affect space weather and satellites. A very bright, prominent layer is seen in the Earth's atmosphere at the altitude where falling snow and hail melt as it is much brighter than atmospheric layers above and below it. Atmosphere's layers, from Earth's surface up to the outer space, are like:
- the troposphere -up to 9 miles (14.5 km). The troposphere is the lowest layer of the atmosphere, as it contains 75 percent of the atmosphere's mass. It is where most of Earth's daily weather occurs with 99 percent of the water vapor found there. Here the air is moving up or down. In that region water vapor, ozone, cirrus clouds and particles such as dust contribute to the amount of radiation or heat allowed in and out of the Earth's
atmosphere system. Temperatures drop progressively down to -61.6°F (-52°C). The altitude of 3.1 mile (5 km) in the troposphere is the region of Earth's atmosphere conducive to the long-range transport of the pollution that is lofted to this altitude. At the upper limit of the troposphere, the lower jet streams are to be found, those impacting commercial jet planes' flights, at a altitude of about 30,000 feet. Strongest and best known jet streams are those near the north and south poles; as these winds blow West to East, they take meandering paths North and South which are determined by their encounter with slow-moving waves, called Rossby waves (Rossby waves on the other hand, might also allow long distance weather connection patterns). That's why jet streams can blow over Florida and other warm places with frigid air. Earth’s equatorial jet stream was discovered after observers saw debris from the 1883 eruption of the Krakatoa volcano being carried by a westward wind in the stratosphere. Later, weather balloons documented an eastward wind in the stratosphere. Scientists eventually determined that these winds reversed course regularly. Jet streams might be linked to the formation of hurricanes. The alternating pattern starts in the lower stratosphere and propagates down to the boundary with the troposphere, or lowest layer of the atmosphere. In its eastward phase, it’s associated with warmer temperatures, and the westward with cooler ones. The pattern is called Earth’s 'quasi-biennial oscillation,' or QBO, and one cycle lasts about 28 months. Gravity waves are considered most likely to be responsible for forcing the QBO to change direction, though they don’t appear to be strong enough to do the job alone. Another type of wave, the gravity inertia waves, which may be hinted to by repeating cloud patterns, also can interfere with the process. The tropopause (9-11 miles (14-18 km)) is a buffer zone between the troposphere and the next main layer. 'Dark lightning', first observed in the 1990s, is a type of electrical discharge within a thunderstorm that
produces 'terrestrial gamma-ray flashes' in regions of the atmosphere with strong electric fields. Fields accelerate electrons to almost the speed of light and they collide into air molecules and produce gamma-rays which in turnproduce electrons and positrons, crashing then into air molecules, with more gamma-rays
- the stratosphere -from 11 to 31 miles (17-50 km). Only the highest clouds (cirrus, cirrostratus and cirrocumulus) are found in the lower stratosphere. Due to the absorption of Sun's ultraviolet radiation, stratosphere is a zone where temperature increases back to +26.6°F (-3°C), or a temperature inversion. Temperature inversion in the statosphere occurs because ozone there absorbs much of Sun’s ultraviolet radiation, preventing it from reaching the surface. It is in the stratosphere indeed that the famous ozone layer is found (at 10 to 25 miles (15 to 40 km) of altitude). The ozone (O3), an unstable form of the oxygen (O2), is a result there of the interaction between ultraviolet and oxygen. It is protecting us from the ultraviolet radiation from the Sun as it is a pollutant in the atmosphere's lower layers or a byproduct of lightning. The air in the stratosphere is flowing mostly horizontally. Ozone-rich stratospheric air may descend into tropospheric air near the ground, bringing ozone to the ground, or a 'stratospheric ozone intrusion.' Low-frequency sound waves exist in the stratosphere. Stratospheric mountain waves are created by winds in the polar vortex. The stratopause (between 31 and 37 miles (50-60 km) is separating the stratosphere from the next layer. Between 40 and 110 miles of altitude, the high atmosphere endure both the influence of winds in the meteorological layer and of solar events
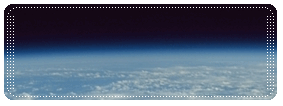 | the Earth's limb, as seen from space, is finely showing the Earth's atmosphere! picture site 'Amateur Astronomy' |
- the mesosphere -from 37 to 53 miles (60-85 km). There, temperature drops back and falls as low as -135.4°F (-93°C). Atoms in the mesosphere are in an excited state as they are absorbing energy from the Sun. Mesospheric or 'noctilucent' clouds, which may be seen sometimes after sunset, are found in that level of the atmosphere. Dusty plasmas are charged particles that can occur naturally in the mesosphere. The mesopause separates the mesosphere from the next layer
- a region stretching 40-110 miles (60-180 km) in altitude is now better known, encompassing the mesosphere and lower thermosphere/ionosphere, or MLTI. That region is where incoming energy from the Sun transforms into the lights of the aurora, where glowing night-time noctilucent clouds appear, and where a dynamic electromagnetic system experiences space weather effects that can interrupt man-made satellites and human communications. Its chemical and thermal balance can change rapidly, mostly from solar inputs, including the Sun's 27-day rotation. Repeating patterns occur at different time scales, from hours to years. It is also a region where temperatures are cooling, and with fewer particles, there is less drag upon satellites orbiting. A dense layer of the ionosphere known as the F-layer, a layer that is particularly variable over the Equator, is impacting upon radio communications as it is so dynamic that it is almost never the same twice. Astronomers now know that the incoming high-energy solar radiation impacting in MLTI is significantly larger than models had predicted. That region is also featuring high-altitude jet stream winds at 60-65 miles (37-40 km) in altitude. Electrojets are structure which circle through the atmosphere around 60 to 90 miles above Earth, near the poles
- the ionosphere -from 45 to 370 miles (75-560 km). The ionosphere is comingled with the very highest — and quite thin — layers of Earth’s neutral upper atmosphere, making this region at the flux between Earth’s conditions and those in space. At 150 to 200 miles (240-320km) above Earth, the air turns extremely thin and these vapor clouds disperse rapidly and follow the winds which can be moving at a few hundred miles per hour there. The ionosphere not only responds to space weather but to Earth's. Vast winds high above Earth’s surface carry energy around the globe and can
modify the ionosphere indirectly by pushing around charged particles in the
upper atmosphere. That motion creates an electric field, which guides the
behavior of particles throughout the electrically charged ionosphere. During nighttime, bubbles of charged gas appear over the equator and tropics, sometimes interfering with radio communications. The ionosphere is a shell of charged particles (electrons and ions), called plasma, that is produced by solar radiation and the impact of its energetic particles, along with the precipitation of magnetospheric particles, which is particles from the magnetosphere, that magnetic shield to the Earth, sometines depositing in the atmosphere below as cosmic rays also play a role. The ionosphere, which hosts a complex system of electric and magnetic fields also, is both shaped by waves from the atmosphere below and changing conditions in space above. As changes in the ionosphere are mostly driven by solar events, energy outside is accumulating until it discharges, not unlike a lightning. The ionosphere also counteracts heating in the upper atmosphere due to the thermosphere, or the overcooling. The ionospheric plasma interacts and co-mingles with the neutral particles of the tenuous upper atmosphere. The upper atmosphere and ionosphere change constantly in response to forces from above and below, including solar events, intense upper atmosphere winds and dynamic electric field changes. The region of the Earth's atmosphere above the ozone layer has the solar strong ultraviolet radiation breaks down neutral molecules, changing them from something resembling the air we breathe into more reactive forms of gas, like atomic oxygen. These reactive compounds in the neutral upper atmosphere produce a faint, global glow, called 'airglow.' Airglow is a red, green, purple and yellow swaths of light, stretching 50-400 miles of altitude, as it occurs when atoms and molecules in the upper atmosphere -- mostly nitrogen and oxygen --, excited by
sunlight ultraviolet, emit light to shed their excess energy. Or, it can happen when atoms
and molecules that have been ionized by sunlight collide with and capture a free
electron. In both cases, they eject a particle of light — called a photon — in
order to be back to their ordinary state. The phenomenon is similar to auroras, but energized by ordinary radiation instead of the solar wind. The tenuous, subdued bubble of light closely encases our entire planet. Breaking those molecules further, sunlight knocks off electrons, leaving a sea of charged electrons and ions which constitutes the ionosphere. The ionosphere thus exists in the same space as the extremely thin neutral upper atmosphere, making Earth's interface to space a unique region where charged and neutral gases coexist. That area responds both to weather pattern from Earth below, and solar space weather above. The ionosphere displays a profile of clumps of plasma, with regions of lower density (bubbles) in the neighborhood of high-density ionization areas. Perturbations or irregularities in the ionosphere come in the form of ionospheric depletions or bubbles, structures that contain fewer electrons, and enhancements or blobs that contain a greater number of electrons, which impacts waves transmission. Blobs can be the direct result of bubbles forming near the geomagnetic equator as they can be observed in regions where bubbles do not extend and can form when bubbles do not. Wave-like thermospheric structures are creating waves in the ionosphere through ion-neutral drag -- a phenomenon called Medium-Scale Traveling Ionospheric Disturbances, or MSTIDs, which create electric fields that can transport energy from the summer to the winter hemisphere and blobs might be the consequence of these electric fields. Ionosphere is where auroras are occurring. Temperature is increasing due to Sun energy and may eventually reach about +3,140°F (+1,727°C). The ultraviolet radiation of Sun, there, is stripping atoms and moleculules from their electrons -'ionizing' them. Many free electrons are found, as a layer of ionized gas, or 'plasma' is produced. The ultraviolet radiation is made of the most energy-charged photons of the solar wind. Ionosphere is reflecting too radio-waves making long distance radio communication possible. A drop in the number of energized particles in the ionosphere at night allows radio waves to reach farther. Layers -- where the plasma is abundant -- and rifts -- opposites were it is less -- in the ionosphere are the cause of radio waves disruption. They are forming suddenly and lasting for several hours, acting like giant mirrors. The height of the ionosphere is varying from daytime to nighttime, with values passing from 600 (960 km) to 400 miles (640 km) respectively, due to a decrease in the ionizing radiation and that charged particles recombine gradually through the night and density drops. The ionosphere is roughly divided into three regions in altitude based on what wavelength of solar radiation is absorbed: the D, E and F, with D being the lowermost region and F, the uppermost. Solar cycles further are influencing that height, with the values weeker during a solar minimum (500 miles -800 km; 260 miles -420 km). The ionosphere parts into the D, E and F regions, in a order of altitude -- D the lowest -- D region absorbs hard x-rays below 56 miles (90km) and reflects AM radio waves, E soft ones (about 65 miles (105km)) and the same, as the F extreme UV (65-373 miles (105-600km)) reflecting shorter radio waves. The electron density of region E is critical in the role played by the ionosphere as far the radio signals journey is concerned, and contributing to the thermal balance of it. E region was so-named by early radio pioneers that discovered the region was electrically charged, and so could reflect radio waves. The E-region can be affected by events implying positively charged nitric oxide. The F region is home to a process called the 'equatorial spread F,' or ESF as these disturbances occur after sunset at latitudes near the equator. Layers E and F are part of the nighttime ionosphere. The E and F regions contain free electrons that have been ejected from their
atoms by the energizing input of the Sun’s rays, a process called photoionization. After nightfall, without the energizing input of the Sun to keep them separated, electrons recombine with the positively charged ions they left behind, lowering the regions’ overall electron density. The daily cycle of ionization and recombination makes the E and F regions especially turbulent and complex as vertical winds in these regions create a tumultuous particle soup that re-distributes the energy, momentum and chemical constituents of the atmosphere. At the base of the ionosphere, charged
particle motions create a global current, along with a one of neutral particles called the 'atmospheric dynamo' moving in loops from the equator to the poles. Such loops are determined by electric fields generated by the
solar activity. Solar soft X-rays brought during a solar flare, are deposited lower in Earth's atmosphere than the extreme ultraviolet radiation and cause almost instantaneous changes in the ionosphere that can disturb radio communications and GPS satellites. X-rays in the Earth's atmosphere near the north and south poles, are produced by electrons raining down into the ionosphere from the Van Allen belts and helped scattered into by low-frequency electromagnetic waves. Lightning burst in the Earth atmosphere create electromagnetic waves that begin to circle around Earth captured between Earth's surface and a boundary about 60 miles up. Some of the waves – if they have just the right wavelength – combine, increasing in strength, to create a repeating atmospheric heartbeat, at a very low frequency, known as Schumann resonance which constitutes a special kind of low frequency wave leaking out into space from
Earth's lower atmosphere, at altitudes of 250 to 500 miles. Response of ionosphere to solar energetic events or even lightnings in terms of propagation of radio waves, or radio degradation, also called 'scintillation,' have its maximum occurring above Africa and generally at low latitudes near the equator. Charged particles of the ionosphere and the neutral particles of the atmosphere directly affect each other as the neutral wind creates piles of neutral gas pushed up against ionospheric density variations. Scintillations are due to the largest disturbances generally at low altitude in the ionosphere at night, themselves due to strong shears in the horizontal ionosphere motions at the base of the ionosphere, places where charged particles flow by each other in opposite directions. During a total eclipse, circular bow waves, or disturbances in the ionosphere's electron density may sped along the path of totality at 300 miles per second. Traveling ionospheric disturbances are sometimes responsible for space weather patterns in the upper atmosphere, and are often linked to atmospheric gravity waves. The ionosphere, generally, is a region of weakly electrically charged gas high above the main body of a planet’s atmosphere. Its shape and density are partly controlled by the internal magnetic field of the planet. As far as Earth is concerned, which has a strong magnetic field, the ionosphere is relatively stable under a range of solar wind conditions. Chimneys of lower density form at the base of the ionosphere and then rise up. Atmospheric Movements down below, closer to Earth, actually have a strong effect too on the ionosphere, with daily cycles and directly linked to Earth's weather. In the layers lower than the ionosphere further, charged particles also exist as they are pushed around by neutral gas. The ionosphere is crucial for modern communications. Low-frequency radio waves bounce off it to travel from one part of Earth to another. Various satellites, including the global positioning system (GPS), send high-frequency radio waves
through the ionosphere down to receivers on Earth. Sunlight heats the atmosphere up at 250 miles above our planet. Ionospheric thunderstorms around 186-373 miles (300–600 kilometers) above Earth occur when the number of electrons in the ionosphere undergoes large and rapid changes, tending to happen close to Earth’s magnetic equator and typically just for a couple of hours between sunset and midnight. A thunderstorm scatters free electrons of the ionosphere and create small bubbles with little or no ionised material. Such bubbles disturb the GPS signals. Counterintuitively, the most energetic solar events are likely to provide a net cooling and shrinking effect on the Earth's upper atmosphere, rather than heating and expanding. That is due to nitric oxide which acts as a coolant at very high altitudes and causing 'overcooling,' which also counteract the tendency of the atmosphere to swell in response to a solar event due to heating, and make it collapse back instead. The temperature of atomic hydrogen, generally, on a other hand, rises when solar activity falls, counter to the behavior of most other neutral molecules in the atmosphere
- the exosphere -from 400 to 800 miles (640-1,280 km) is the ultimate layer of Earth's atmosphere. It begins where the ionosphere ends and is part of Earth's outer environment which progressively merge into the outer space. Hydrogen and Helium are still present there and are the main components. They are found at extremely low densities however. Earth's exosphere encompasses Earth's magnetosphere as soft X-ray emissions exist there which could result from the 'charge-exchange phenomenon', a result of solar wind's interaction with interplanetary neutral atoms. Birkeland circuit is composed supersonic plasma jets linked to vast sheets of electric current in the upper atmosphere as the theory that there were huge electric currents powered by solar wind and guided through the ionosphere by the magnetosphere, was postulated by early 20th century by Norwegian scientist Kristian Birkeland. They were confirmed in the 1970's after the advent of satellites. Birkeland currents are associated with large electrical fields and the Birkeland circuit is a organising feature of currents for the coupled magnetosphere–ionosphere system. Currents are stronger in the northern hemisphere and vary with the season as the correlated electrical fields occur where upwards and downwards currents connect through the ionosphere and are at they strongest in winter. Those electric fields in turn generate supersonic plasma jets named 'Birkeland current boundary flows' and can drive the ionosphere up to 10,000 degree C and change its chemical composition. They also cause the ionosphere to flow upwards to higher altitudes where additional energisation can lead to loss of atmospheric material to space
->Plasma Bubbles in the Upper Atmosphere
Late studies by NASA have shown that plasma bubbles form at night in the thermosphere and ionosphere, with a mix of plasma and electrically neutral gas becoming instable after sunset. During the daytime, radiation from the sun creates plasma by tearing electrons from atoms and molecules in the thermosphere and ionosphere. When night comes, there is no solar radiation anymore as the charged particles recombine back into electrically neutral atoms or molecules again. The recombination happens faster at lower altitudes, because there are more heavy charged particles (molecular ions) there, and they recombine more quickly than charged particles made from single atoms. More rapid recombination makes the plasma less dense at lower altitudes and leads to more instability still. The equatorial regions are especially turbulent further because the plasma bubbles are suspended on Earth's magnetic field, which is horizontal over the equator. The boundaries of such bubbles, particularly at the equator, leads to satellites' communication and navigation signals to be interrupted. Plasma bubbles form between 53 and 370 miles of altitude. Upwards motion of clouds which may reach 600 miles wide, may attain hundreds of yards per second, causing electromagnetic turbulences. Such bubbles occur preferentially at some seasons
Website Manager: G. Guichard, site 'Amateur Astronomy,' http://stars5.6te.net. Page Editor: G. Guichard. last edited: 9/21/2018. contact us at ggwebsites@outlook.com