CONTENT - A description of our Sun which is both studies in terms of its internal structure and the miscellaneous energetic events he triggers |
|

The Sun is certainly the most perfect sphere, like an object, in the solar system, due to its intense gravity, with an oblateness of a mere 8.01 milli-arcseconds in excess in terms of apparent diameter. The years of high solar activity however are turning the Sun oblate somewhat due to 'supergranules' -large convection cells- emerging near the Sun's equator and adding a width of 10.77 milli-arcseconds to the Sun's apparent diameter. Sun has a layered structure
->Everything In and On the Sun Linked to the Inner Dynamo!
Massive currents of electrically-charged gas (plasma) circulate in patterns in the inner layers of the Sun, which yield the solar dynamo, which in turn gives to the Sun's powerful magnetic field. Almost all solar activity from sunspots to solar flares is regulated by that inner dynamo, as the only way to appreciate how it works is to use the 'heliosismology' consisting into monitoring the waves created by the inner motions of the Sun and coming to 'ring' against the surface. The Sun's complex magnetic field is mostly made up of closed loops of magnetic field with both ends anchored in the Sun. The Sun's less-common open field lines migrate between the Sun's north and south poles over the course of the 11-year solar cycle, during which the overall magnetic field reverses in polarity. The solar magnetic field plays a vital role in dictating the structure of the Sun's atmosphere, and acts as a conduit for mass and energy to flow into the solar corona and solar wind
- The Sun's core is the place where our star is generating its energy by the mean of fusion nuclear reactions which transform the hydrogen into helium. The conversion of hydrogen into helium is the source of 99% of the Sun’s energy.
The multistep process begins when the star’s hot, dense core squeezes two
protons together to form deuterium, a heavy isotope of hydrogen with a nucleus
made of one proton and one neutron. One of the fused protons then transforms
into a neutron, a process that releases a neutrino and a positron (the
antimatter counterpart of the electron). Three reaction sequences are collectively known as the proton-proton (pp) chain. The chemical composition of the Sun is not well established like its metallicity, the abundance of all solar elements heavier than helium). At the core, the temperature is 27 million degrees Farenheit (15 million degrees C), with a density of 150 g/cm3 (a strong one!). The core has a radius of 108,700 miles (175,000 kilometers); we are 323,200 miles (520,000 km) under the surface. The energy is now going to ascend through the diverse layers of the Sun as it will eventually leave it under the form of light. Seismic g-mode waves in our Sun revealed in 2017 that the solar core is rotating some
four times faster than the solar surface. The Sun is constantly
ringing with sound waves because of the continuous convection of solar material going on beneath the surface. Such those g-mode, or gravity-mode waves are fluid waves where buoyancy acts as the restoring force but they are hard to detect and they were searched during over 40 years. Sound waves, also called pressure waves, or p-waves, on the other hand, are easy to detect on the solar surface. The imprint of these g-waves suggests that the solar core is rotating once every
week, nearly four times faster than the Sun’s surface and intermediate layers, which have rotation periods anywhere from 25 days at the equator to 35 days at the poles. A new set of questions has been opened now for solar physicists, such as how differently-rotating layers
of the Sun interact. G-mode waves had already been detected in other stars
- The energy, through a first layer -the radiative zone, is leaving the core under the form of a radiation -in that case light. The radiative zone extends from minus 323,200 to minus 124,300 mi (520,000 to minus 200,000 km); the temperature drops to 3.6 million degrees F (2 million degrees C) and the density passes to 0.2 g/cm3
- The energy then is passing through an intermediate layer -the 'tachocline', which is thought to yield the magnetic field of the Sun. It reaches a second layer -124,300 mi (200,000 km) below the surface- where elements are beginning to re-combine and where radiation becomes matter. It traps the heat and by the mean of convection, it's heading to the Sun's surface. That's why the layer is called the 'convective zone.' The rotation of a star and the flow of gas in its interior both play a role in producing its magnetic field. The rotation of the Sun and similar stars varies with latitude as well as in depth below the surface. Another factor in the generation of magnetic field is convection. The process of convection in a star distributes heat from the interior of the star to its surface in a circulating pattern of rising cells of hot gas and descending cooler gas. Convection occurs in the outer third of the Sun, while the hot gas closer to the core remains relatively still. There is a difference in the speed of rotation between these two regions. Many astronomers think this difference is responsible for generating most of the magnetic field in the Sun by causing magnetic fields along the border between the convection zone and the core to wind up and strengthen. Since stars rotate more slowly as they age, this also plays a role in how the magnetic field of stars weakens with time. The interaction, on a other hand, along the convection zone-core boundary might not dominate the generation of magnetic field in stars like our Sun, since low mass stars studied have shown they lack such a region of convection and yet their magnetic properties are very similar. For stars much less massive than the Sun, convection occurs all the way into the core of the star. That outer third of the Sun had a different rotating speed that the rest of Sun's as the shear arising from this difference could be responsible for generating the Sun's sunspot activity cycle
- The visible surface of the Sun is called the 'photosphere'. It is 62 mi (100 km) thick. It is this layer which is mainly accessible to observation. Compared to visible light, the Sun’s extreme ultraviolet output is highly variable. The temperature is now 10,400° F (5,760° C) as the density is 0.0000002 g/cm3 -1/10,000th of the air's density. The photosphere is the realm of sunspots. Sunspots are now believed to be places where magnetic fields are preventing the incoming heat to reach the solar surface. Hence the plasma becomes cooler. Hence heavier, and it sinks (at 3,000 mph -4,800 km/h). The sunspots are the places of this event. Sunspots are darker because they are cooler than their surroundings, as the sinking process holds together the magnetic lines of same polarity at the same time (sunspots work in pairs, each sunspot has one polarity, either positive or negative; the magnetic fields of same polarity found in one sunspot should tear it apart). The sunspots activity is ruled by a 11-year cycle. The solar activity -and the number of sunspots- is reaching a maximum, then a minimum
Plasma tornados are created by magnetic fields spinning the plasma. Giant waves of plasma travel around the Sun at up to 3 million miles per hour and named EIT waves after a instrument of the same name on the SOHO spacecraft. They might be driven by coronal mass ejections. Moving about at solar mid-latitudes, are large-scale circulation patterns called Meridonial circulation. A NASA Marshall Center study presented in 2003 found that the Sun's 11 years cycle might be driven in part by a 'giant circulation system' 125,000 mi (201,100 km) below the solar surface. Compressed gases would start at the poles and move to the equator at a depth of 125,000 mi (201,100 km) and at 3 mph (4.8 km/h). They would then move back to the poles, in the upper layers this time, and at 20-40 mph (32-64 km/h). This current would transport a magnetic field. When the 11-year cycle is longer than usual, the flow is slow. When the cycle is shorter, it's fast. In this later case, some magnetic field is piling up at the poles as it's then transported to the equator by the depth current. It's moreover amplified, as it yields a cycle with a strong activity. The same study showed that granules of the Sun are pillow-shaped and that faculae are accumulation of magnetic fields against the walls of the granules. A important energy too is released by the rupture of granules which rearrange into new intergranular lines as, even with a calm Sun, horizontal vortices are seen traversing the granules, a magnetic tubes and convective lines are also part of the energy in those regions. Granules are convective features resulting from hot, less dense material rising as more dense, cold one is descending. Granular cells have a size of 620 to 930 miles and a existence of several minutes only. The solar magnetic field, on the other hand, is flipping each 11 years, with its poles flipping from one pole of the Sun to the other (it's currently inverted, with the magnetic south pole near the Sun's north pole, and reciprocally) as the scientists suspect that the temperatures at the southern pole of the Sun might be different from the ones at the north pole. Sun's magnetic reversal may be inferred from the disappearance of polar coronal holes
- Beyond the photosphere is lying the 'chromosphere'. It is an irregular layer where the temperature passes from 10,400 to 36,000° F (5,760-20,000° C). This high temperature makes that the hydrogen turns red. Hence the name of the layer ('chromos', in Greek, means 'color'). It is this process which is called the 'H-alpha emission'. The chromosphere is the zone of the prominences. Prominences -called filaments when seen against the Sun instead of over the horizon- are unstable clouds of solar material suspended above the solar surface by Sun’s complex magnetic forces. Prominences are the plasma of Sun -a electrified gas in which positively (ions) and negatively (electrons) charged particles have separated, forming a superhot mix responding to solar magnetic fields in the Sun's atmosphere- sculpted by magnetic fields and which then bursts through the surface into the solar atmosphere. When the magnetic loops reach up into the solar atmosphere, huge streams of plasma are attracted to fill them for weeks or months. When a prominence starts to collapse, the gas drains down back to the surface through those magnetic fields. A few spectacularily large prominences occur each year. They most often break apart after a few days. When a solar prominence falls back to the solar surface, it may trigger a magnetic reconnection -- the brutal reorganization of the lines of magnetic filed -- when forcing upon magnetic likes on the way. That's the difference between a forced, and a spontaneous reconnection. The spontaneous type is the more frequent. These solar eruptions may be seen rising on the limb during eclipses or through an H-alpha filter. Such events are magnetic, arc-shaped tubes of hot gas as he solar plasma is a gas in which the negative electrons move freely around the positive ions, forming a powerful mix of charged particles. Each burst of plasma licks out from the surface only to withdraw back into the active region, a dance commanded by complex magnetic forces above the Sun. Important filaments and prominences are often associated with coronal mass ejections (CMEs; see below) as smaller ones are linked to the boundaries between the sunspot active regions. Prominences have a relative low temperature compared to the background. They appear bright when seen on the limb as they appear dark when they are seen on the disk. In this case they are termed 'filaments'. The chromosphere may be considered the atmosphere of the Sun or its gaseous envelope. 90 percent of loops forming above the solar surface into the chromosphere or the corona, might have one foot located into a relatively intense magnetic field as the other resides inside the photosphere. Kelvin-Helmholtz instability as it kicks up hot plasma, by interval triggers lengthy waves on the surface of the Sun appear moving at speeds as high as 4.5 million miles per hour and periods in the range of 30 to 200 seconds, with effects into the corona, accelerating the solar wind, triggering remote eruptions, and
delivering energy and information between different parts of the solar atmosphere. The chromosphere further features, in its lower area, a cooler layer at 7,232° F (4,000° C). Such a cool layer also exists at other Sun-like stars
- A second external region -the 'transition region'- is then found. It's a very thin region which is produced by the heat which is descending from the solar corona into the chromosphere. The region is quickly passing from 40,000 to 1,800,000° F (22,000-1 million degrees C). The hydrogen becomes ionized (it's difficult to see), and the zone mainly produces light in the ultra-violet. Such a light is coming from the ionized carbon (C IV), the ionized oxygen (O IV) and the ionized silicon (Si IV), as it's observable from space only. The transition region may harbor sunspots or solar flares
- At the farthest from the Sun's surface at last, a last region is found. It's the 'corona', or 'solar corona'. The corona is a kind of external atmosphere to the Sun. English astronomer Francis Baily by 1842 was the first to suggest that the hazy 'corona' seen during a total eclipse was the Sun's atmosphere. Visual evidence that the staggeringly hot material filling the corona has definite structure on fine scales, rather than being a homogenous soup of particles, was brought lately as it was imaged constituted of fine strands of million-degree solar material. The question of the corona's heating arose by 1942 from Swedish astronomer Bengt Edlen who first measured corona's temperature and found it was much hotter than the solar surface. Swedish physicist Hannes Alfvén next year proposed the existence of wave in electrically conducting fluids, a mechanism which could be applied to the Sun. U.S. astrophysicist Eugene Parker who, in 1958, had theorized that the solar wind was linked to the solar corona, proposed by 1988 that frequent, small eruptions on the Sun called 'nanoflares' was responsible for the coronal heating. Parker's theory and the one of Alfvén (the coronal heating is yielded by the dissipation of the waves which bare his name) are both preponderant -- and opposed -- theories regarding the coronal heating. Scientists aren't sure how the temperature inversion which exists into the corona happens. The story of the coronal heating problem begins with a green spectral line observed during an 1869 total eclipse. The green line observed in 1869 didn’t correspond to any known element. Scientists thought perhaps they’d discovered a new element, and they called it coronium. Not until 70 years later did a Swedish physicist discover the element responsible for the emission is iron, superheated to the point that it’s ionized 13 times, leaving it with just half the electrons of a normal atom of iron. And therein lies the problem: scientists calculated that such high levels of ionization would require coronal temperatures around 2 million degrees Fahrenheit — nearly 200 times hotter than the surface. One theory proposes that magnetic waves called Alfvén waves steadily convey energy in the corona where it is then dissipated as heat. Coronal waves are one possible source of coronal heating. Alternatively, micro explosions, termed nanoflares -- too small and frequent to detect individually, but with a large collective effect -- might release heat also. The lower part of the corona, is thought to hold the keys to several solar mysteries, from the acceleration of the solar wind, to the birth of explosive clouds of solar material called coronal mass ejections, to the mysterious heating of the corona as a whole. Since April 2015, astronomers think the heating mechanism depends on regular, but intermittent explosive bursts of heat, rather than on continuous gradual heating, or a process called nanoflares. A good explanation appeared by 2019 is that Sun's magnetic waves grow in strength and become hotter than the surface of the Sun itself as they leave the interior, due to the formation of a 'acoustic resonator,' in which significant changes in temperature between the solar surface and its outer corona create boundaries that are partially reflective, acting to trap the waves, allowing them to intensify. As of June 2014, the corona was found even larger than thought, extending out some 5 million miles above the Sun's surface -the equivalent of 12 solar radii- as giant
solar storms or coronal mass ejections can create ripple effects felt through the corona. Beyond that boundary, however, solar material into the steady solar wind. During a total eclipse, the solar corona is visible like a radiating zone, stretching far from the occulted solar disk. The corona is an extremely hot region 1.8 million degrees F (1 million degrees C) or more, where most of the elements, the heaviest excepted (and they are just a few of them like the iron or the calcium) are ionized. The polarization of the ultraviolet light in the solar corona -or its restriction to one direction- can be correlated to the intensity and direction of the magnetic field. Scientists generally agree that energy in the roiling magnetic fields of the Sun must transfer energy and heat up into the atmosphere -- and the solar wind -- but the exact details of that process are still debated as the most recent studies hint to that heating must be quick and impulsive. The general aspect of the corona varies according to the 11-year cycle. To observe the corona outside the period of an eclipse, one uses a coronographs, which is a device which artificially occults the solar disk), or one observe from space, in the X-rays. Due to its high temperature, the corona is very visible in this range of the wavelengths. On the other hand, as the photosphere is barely emitting in this range, the corona, in the X-rays, is even visible through the solar disk! Iron at very high temperatures is the largest contributor to corona. The heating of the corona is still ill explained. It is likely to be related to the twisting and snapping of magnetic field lines sending energy rippling through the atmosphere and out into space under the form of solar storms. A part of the heating is provided due to turbulences occurring in the chromosphere and generating so-called 'Alfven waves'. The Alfven waves are created when convective motions and sound waves push magnetic fields around, or when dynamic processes create electrical currents that allow the magnetic fields to change shape or reconnect. The Alfven waves last about several minutes. Loops of hot gas that arch high above the solar surface are making up too the corona. Those loops are made up of smaller, individual magnetic tubes or strands as the sudden burst of so-called nanoflares, which are small and sudden bursts of heat and energy, happen within those tubes. The ultra-hot plasma which is directed into the corona by the giant loops is cooling out very quickly and is very difficult to detect thus as the gas then rains down back to the solar surface, alimenting a kind of looping process between the surface and the corona! Some nanoflare activity, like the one responsible for the heating of the solar corona, is seen too in the Sun's active regions, yielding changes in the X-ray and ultraviolet (UV) radiation that happen as an active region evolves. Depending on how many and how energetic these are, nanoflares may be the missing piece of the puzzle to help understand what seeds the cascade that causes a much bigger flare, or to explain how the Sun transfers so much energy to its corona. It is not completely known whether nanoflares are driven by the same mechanisms as larger solar flares and whether nanoflares are mini-versions of this process or are just small heating events is currently unknown. Giant magnetic coronal loops -some over 100,000 miles long- play a fundamental role in governing the physics of the corona. Such bright, thin strands are interacting with Sun's magnetic field. Sun’s small-scale magnetic fields themselves could contribute, in principle, sufficient energy to heat the corona. Giant, sleak plumes of gas known as spicules and as long as Earth zooming up from the Sun's surface at 150,000 miles per hour are one of several phenomena known to transfer energy and heat into the corona. At any given moment 10 million transient spicules burst from the Sun’s surface, reaching up to 6,000 miles before collapsing. They also originate from islands of tangled magnetic lines managing their way to the surface, the buoyancy of which is provided by a mix of neutral and electrified particles. When the magnetic lines snap, they create spicules and release both plasma and energy. The process of spicules also generates Alfvén waves which, along with forcing plasma out, plays a role to heating the lower layers of the corona and propelling the solar wind. Spicules in visible light are seen to send large masses of plasma through the photosphere as generally the hundred thousand degrees major part of the gas they spew is falling back as the million degrees, hottest part of it remains aloft and falls later on. Erupting gas flowing by stationary gas in the Sun's atmosphere on a other hand could give rise to Kelvin-Helmholtz instabilities or waves the size of the United States. Kelvin-Helmholtz instabilities occur when two fluids of different densities or different speeds flow by each other. In the case of
the solar atmosphere, which is made of a very hot and electrically charged gas
called plasma, the two flows come from an expanse of plasma erupting off the
Sun's surface as it passes by plasma that is not erupting. The difference in
flow speeds and densities across this boundary sparks the instability that
builds into the waves. Despite the Sun's corona which is permeated with magnetic fields which can have a stabilizing effect, such waves have indeed been observed there. Waves also can cascade down to smaller forms of turbulence too as the friction created by those could help add heating energy to the corona. The detailed mechanics however of coronal heating remain currently unknown. Tails of comet which come to graze the Sun too close are rapidly ionized by losing electrons in that hot environment and they can act as a tracer of the complex magnetic system higher up in the corona. Certain kinds of solar seismic waves are also channeling upwards into the chromosphere, and whence into the corona. Instead of traveling straight upwards through the Sun, the waves veer off, taking a curved path through the atmosphere. When waves travel upwards, some may reflect back downwards, or contribute to heating of the corona. 'Coronal rain' is constituted of giant globs of plasma that drip from the Sun's outer atmosphere back to its surface. Coronal dimmings are linked to CMEs
- Once the energy of the Sun has journeyed through the corona, here begins the realm of the solar wind, and the interplanetary space
->The Solar Magnetic Field Makes a Difference at the Solar Poles The ESA-NASA Ulysses spacecraft found again -like in 1995-1996- that there is a difference of a 7 to 8 percent in temperature between a cooler north pole and a warmer south one, with a difference in temperature of the order of 80,000° F(44,000° C). It seems related to the structure of the magnetic field as that difference has followed the 11-year cycle of the flip of both solar magnetic poles. That's of importance as the solar wind emanates from the solar poles
The Ulysses saw another tricky observation, as a solar storm which emerged from a sunspot region at the equator was funneled in such a way that the craft was hit as it was overflying the south pole, as the solar wind emanates from the Sun's poles. That flow from the poles in terms of the solar wind may have the latter spill down to the Sun's equator, or to be confined to the mid-latitudes only
->How Solar Science Evolved
As astronomer Thomas Harriot first saw sunspots through a telescope in 1610, the study of the Sun really took off about 130 years ago following Dutch astronomer Pieter Zeeman’s discovery that a magnetic field, or a field of force generated by electrical currents, alters some spectral lines. Within a decade, American astronomer George Ellery Hale used Zeeman’s discovery to demonstrate that sunspots contained strong magnetic fields. After World War II, when there was a growing recognition of solar activity’s influences on radio frequency propagation in Earth’s upper atmosphere. Scientists used leftover German rockets to soar above Earth’s atmosphere to measure emission in wavelength ranges absorbed by atmospheric gases, and found that the Sun's ultraviolet radiation varied wildly from year to year. Such rocket missions were limited however to five to 10 minutes in duration. With the space age, NASA developed spacecraft called the Orbiting Solar Observatories (OSOs) to study solar activity, with eight spacecraft launched between March 1962-June 1975, making solar science over a complete solar cycle of 11 years. The concept of space weather was found in 1946 by U.S. physicist Scott Forbush when he detected incoming energetic particles spiking not long after a solar flare. Coronal mass ejections, as far as they are concerned, had been predicted in the 1960's and they were first observed by December 1971 from a orbiting space observatory. As soon as by 1859, English astronomer Richard Carrington made a link between a rare solar white flare, and northern lights seen down to the island of Cuba, or telegraph failure (that was called the Carrington Event). Helioseismology also came in the early 1960's, discovering global oscillations (or pressure waves) occurring at the solar surface and helping to probe solar interior as scientists suspected for a while sound waves to also heat the corona. On Skylab, launched by 1973, astronauts operated a battery of telescopes to learn more about variability of the extreme ultraviolet and X-ray emission from the corona as their mission unfortunately showed that a human-operated telescope wasn’t very effective as they nearly always missed the beginning of flares. In 1980s the Solar Maximum Mission improved upon by using automated flare detection to digitally coordinate all the instruments to direct to the flare within a fraction of a second as such early missions laid the groundwork for missions like SOHO. NASA-ESA SOHO studied the internal structure of the Sun, its outer atmosphere and the origin of solar wind. When SOHO was launched on Dec. 2, 1995, the field of heliophysics was still lacking knowledge about those elements. At the time, it was thought that solar flares were the primary Earth-effective solar event, in part because they were the most commonly-observed as SOHO's coronograph allowed to CMEs, a major piece of the space weather puzzle, and much more common—and more variable. SOHO had the Sun seen like a dynamic object as the satellite provided the basis for more than 5,000 scientific papers and yielded the recognition that we live in the extended atmosphere of a magnetically active star. Solar missions, on a other hand, like NASA’s Solar Dynamics Observatory (SDO), NASA’s Solar and Terrestrial Relations Observatory, and NASA’s Interface Region Imaging Spectrograph, or JAXA/NASA’s Hinode have been shaped by SOHO's discoveries. NASA currently flies, for example, multiple spacecraft about the Sun, providing a range of different observations. Astronomers also are using background low frequency sounds of the Sun to study it, from solar flares to coronal mass ejections, or the layers of the Sun
Far from the still, whitish-yellow disk it appears to be from the ground, Sun sports, in the non-visible wavelengths, a dynamic surface and exterior, with twisting, towering loops and swirling cyclones that reach into the solar upper atmosphere. Sun indeed is a giant magnetic star, made of material that moves in concert with the laws of electromagnetism. Scientists are not sure exactly where in the Sun the magnetic field is created. It could be close to the solar surface or deep inside the Sun or over a wide range of depths. As the Sun is made of plasma, a gas-like state of matter in which electrons and ions have separated, creating a super-hot mix of charged particles, when charged particles move, they naturally create magnetic fields, which in turn have an additional effect on how the particles move. The plasma in the Sun, therefore, sets up a complicated system of cause and effect in which plasma flows inside the Sun churned up by the enormous heat produced by nuclear fusion at the center, create the Sun's magnetic fields, a system known as the solar dynamo. Magnetic fields are revealed through loops and towers of material in the corona as footprints on the Sun’s
surface, or photosphere, of these magnetic loops can be more precisely measured
using an instrument called a magnetograph, which measures the strength and direction of magnetic fields. The solar magnetic system is known to drive the approximately-11-year solar activity cycle as, with every eruption, the sun’s magnetic field smooths out slightly until it reaches its simplest state. After that minimum, the Sun’s magnetic field grows more complicated over time until a new peak. At solar maximum, the magnetic field has a very complicated shape with lots of small structures throughout which are the active regions we see. At solar minimum, the field is weaker and concentrated at the poles, a very smooth structure that doesn’t form sunspots. A comparison, in terms of the relative complexity of the solar magnetic field between January 2011 and July 2014 is showing that, by January 2011, three years after solar minimum, the field is still relatively simple, with open field lines concentrated near the poles. At solar maximum, in July 2014, the structure is much more complex, with closed and open field lines poking out all over – ideal conditions for solar explosions. Rossby waves also exist on the Sun, those waves linked to any rotating fluid, which likely are a link between surface's fluid motion and magnetic fields. They could help scientists to better predict the formation of sunspots and the eruption of solar flares. Hot, bright features called brightpoints in the Sun’s corona can be used to track the roiling motions of material deeper in the solar atmosphere and they are linked to increased magnetic activity. Solar Rossby waves flow under the surface and are thought to drive space weather as brightpoints may serve as a clue, linking how the solar cycle leads to increased numbers of solar flares every 11 years. Rossby waves couple activity happening on instantaneous timescales with things that are happening on decadal and longer timescales. Flares and coronal mass ejections that may appear random are probably governed at some level
->Do the Major Sun-Earth Interactions Occur when Both the Solar and the Earth Magnetic Field Have a Same Polarity?
One thought until now that the major interactions between the solar events and the Earth's magnetosphere did occur when both the Earth's magnetic field and the Sun's one featured an opposite polarity. Recent data, in 2008, by NASA's Themis mission are showing that it might well be the opposite which occurs! The most important breaches into the Earth's magnetosphere might occur when the Earth's magnetic field and the Sun's one have a same polarity. That would constitute a major discovery!
->Flares, CMEs, Proton Storms, Geomagnetic Storms
Taken together, flares, CMEs, and solar energetic particles constitute space weather. The most usual mechanism of a solar event is that a flare explodes from the magnetic field lying over a region of sunspots, unleashing visible light and X-rays. This translates into a Coronal Mass Ejection (CME), with a large amount of solar material expelled into the interplanetary space. CMEs hold charged protons, electrons and heavy ions. As the CME moves, taking some days to reach Earth, it plows through the 'usual' solar wind, accelerating protons to high energies and pushing them ahead. Such particles are termed 'solar energetic particles' (SEPs) as they thus are streams of very fast protons. CMEs may be interspersed into the solar wind like denser clouds of solar wind material. The proton storm then hits Earth, as the CME too, trigerring auroras and bringing potentially harmful radiation to spacecraft and astronauts. Even a particularly fast stream of solar wind may reach so. Mastering the 'solar weather' is of importance for the satellites in orbit around Earth as it will be more important still for the renewed presence of man at Moon, and further to Mars. Astronomers now mostly do not separate flares and CMEs anymore as they better say 'Solar Eruptive Events' instead as a same burst of energy creates both kind of events with part of the energy shooting into the sky and turning a CME and part of the material driven down to the Sun's surface and appearing as the flare. The magnetic field lines on which energetic particles from a flare travel curve from the Sun
to Earth unlike CMEs, which travel in straight lines. Energetic particles were magnetically connected to Earth even though, for example, the associated CME may miss us. Such protons thus are reaching Earth much more quickly than the CME as it even happens that they hit a CME preceding them and create a shock wave there. Solar flares and other energetic events from the Sun are also producing gamma rays by accelerating
charged particles, which collide and interact with Sun's
atmosphere and surface. The area on the right side of the solar disk, generally, has a almost direct magnetic
connection to Earth as the Sun's magnetic field lines curve
as they extend away from the sun in conjunction with Sun's rotation. NASA stated by late 2015, that a 12 percent chance of a giant solar flare is extant in the next 10 years, able to bring global, EMP-type damages on Earth. A geomagnetic storm is fast-moving material coming from the Sun -either high-speed solar wind streams, or CMEs- colliding with Earth's magnetic field and sending it oscillating
->More About Flares and CMEs!
Two main types of explosions occur on the Sun: solar flares and coronal mass
ejections. Coronal mass ejections and solar flares are, at our Sun, a expression of the reconnection phenomenon, like the one seen in the Earth's magnetosphere, a process which can affect plasma, or space filled with electrically charged particles and electric and magnetic fields. Unlike the energy and X-rays produced in a solar flare -which can reach Earth at the speed of light in eight minutes and be constituted originally with light, energy and X-rays- coronal mass ejections are giant clouds of solar material that take one to three days to reach Earth. The strongest flares are almost always correlated with CMEs. A dramatic magnetic power struggle at the Sun’s surface determine whether a flare is followed by a CME. A magnetic cage prevents the growing of a twisted magnetic rope associated with the onset of a CME. The rope however may be sufficiently strong to trigger a flare. Both eruptions are created when the motion of the Sun’s interior contorts its
own magnetic fields. Like the sudden release of a twisted rubber band, the
magnetic fields explosively realign, driving vast amounts of energy into space. That constitutes a kind of magnetic reconnection. Strong electric fields are generated that produce a large force on charged particles and, in the ionized gas of the Sun’s atmosphere, this process sends electrons and ions flying at speeds approaching the speed of light, causing them to release high-energy gamma rays. This phenomenon can create a sudden flash of light, or a solar flare. Flares can last minutes to hours (particles accelerated by a CME on the far side of the Sun somehow may reach around to produce a gamma-ray glow on the side of the Sun facing Earth). Some of the energy released in the flare also accelerates very high energy particles that can reach Earth in tens of minutes. The flare is like the muzzle flash, which can be seen anywhere in the vicinity. The
CME is like the cannonball, propelled forward. Traveling over a million miles per hour, the hot material of CMEs called plasma takes up to three days to reach Earth. The differences between the two types of explosions can be seen through solar telescopes, with flares appearing as a bright light and CMEs appearing as enormous fans of gas swelling into space. Solar energetic particles (SEPs) are the streams of high-speed particles blasted from the Sun from narrow flares or wide coronal mass ejections (CMEs). A SEP event typically occurs every couple weeks. The young Sun was more active than nowadays frequently -perhaps even daily- blasting out intense bursts of radiation. As both flares and CMEs can yield disturbances at Earth under the form of radio ones, or magnetic ones respectively, a fleet of NASA heliophysics observatories in space are always on the watch for these explosions as the U.S. NOAA’s Space Weather Prediction Center runs simulations
and can make predictions about when a CME will arrive at Earth, then alert appropriate groups
The Sun is an active body. Magnetic fields emerging from below the surface of the Sun are the essential factor to solar events. The Sun is expelling, through various, usual or unusual events, a flurry of elements and radiation into the solar system. Latest studies might hint to that even small solar events are affecting large regions of our Sun through the dispersion of magnetic instabilities and waves. Solar radiation storms, those bursts of incredibly fast-moving protons and electrons resulting from solar events are solar-accelerated particles can get up to 80 percent of the speed of light. The Sun is working on a 11-year cycle that is that each 5-6 years the activity of the Sun is reaching a peak, as 5-6 years later, it's reaching a low. Generally, it may be considered that it's the sunspots which are at the origin of solar flares, coronal mass ejections, and an intense UV radiation. A 2003 study is showing further that the most unusual and energetic solar events, the Coronal Mass Ejections (CMEs) are related to a cleansing of the Sun's magnetic field. CMEs are increasing at the moment of the peak of the 11-year cycle as does their speed (during last peak, there were more than a thousand CMEs at the Sun). CMEs do not peak however until 2 years after the solar peak. During about the same duration, the solar magnetic field is reverted, that is the North pole of the dipole become the South pole and reciprocally. During these two years, CMEs are polar, occurring at one pole, then at the other two years later. 'Solar braiding,' or energy transfer from Sun's magnetic field to corona is showing how the evolution of the field explain Sun's working and any events like flares. At less of 1 percent of the solar system's mass, the whole of the planets in the solar system, look like they have a influence, in terms of their configurations translates into gravitational effects, on the solar acticity as a whole as they modify the circulation of plasmas, hence the solar global magnetic field and the activity of the Sun along a solar cycle
All the various sorts of the solar outflow are interacting with the planets of the solar system, especially with Earth. Planets are protected from the solar wind, particles, and radiation by a magnetosphere, which is a tear drop-shaped magnetic field extending on the far side of the planet. The most known interaction between the solar events and the planetary media are the auroras. Some solar particles are managing to reach the poles of the planets, where they are making the atoms in the upper atmosphere glow. Auroras, at the Earth, are usually occuring from 60 miles (96 km) to several hundred miles (several hundred km) high, as some may occur to more than 500 miles. Most recent studies, generally, are showing that there are far more short-term changes in solar activites than previously thought
->Another Feature of Sun?
A study of the Sun by the Japanese craft Hinode has spotted that powerful X-ray jets are expelled from the solar surface hundreds times a day, propeling American continent-sized blobs of hot gas at speeds of 2 million miles per hour (3.2 million km/h). Such jets were already known, as the new craft allowed to see that they are occurring at an important pace of about 240 daily. They are not linked to any place of the surface, occurring about anywhere there. It looks like they are triggered by reconnection events similar, albeit much smaller, than those which power the solar flares, at about a thousand times less than a typical M-class flare. They are scaled-down filamanets. Those X-ray jets might well represent up to between 10 and 25 percent of the input of the Sun into the solar wind. The X-ray jets might too provide for the heating of the corona which remained unclear until now, by throwing there 'Alfven waves' which crack there like a whip and heat the gas
- The Solar Wind. The solar wind is a stream of electrons and protons forming a heated gas called a plasma which
zoom out from the Sun. As it approaches Earth, the solar wind is gusty and turbulent as near the Sun it is structured in distinct rays. That transition from defined rays in the corona to the real thing was better defined by NASA's STEREO observatory by 2016. As the plasma travels further away, the magnetic control of the Sun fades, which forms the boundary defining the outer corona, the 'very edge' of the Sun. Eventually, the material starts to act more like a gas, and less like a magnetically structured plasma. Scientists had hypothesized before that magnetic forces were instrumental to shaping the edge of the corona. Thence, material from the corona streams out into space, filling the solar system with the solar wind. Solar wind is a constantly-flowing stream of particles and magnetic fields. As this solar wind travels it carries along embedded
magnetic fields, which can affect charged particles and other magnetic fields
they encounter along the way. The solar wind is originating in the solar corona, this outermost part of the Sun. The solar wind
originates in a gentle way, evaporating from funnels of solar material that are
rooted in strong magnetic field regions called networks on the Sun's surface. The solar wind's speed is much higher than one would expect as some of the fastest solar wind streams are accelerated in coronal holes, areas on the Sun where the magnetic field is open to interplanetary space. Areas of weaker
magnetic fields and less dense material in the solar atmosphere, called coronal
holes, are typically thought to be a source of the solar wind as magnetic field lines extend out into space and don't return to the Sun, yielding a open magnetic field. It was the finding that comets' ion tails always point away from the Sun which eventually led in 1958 to the discovery of the solar wind, with its magnetism and velocity pushing the comet's tail outwards. The solar wind is accelerated in the Sun's corona, the hottest part of its
atmosphere, so its atoms have been ionized or stripped of many of their
electrons. The Solar wind is a plasma, a material of charged particles behaving like a fluid. The solar wind is an electrically conducting gas called plasma where matter has been torn apart into electrons, which are relatively light, and positively charged ions, which are thousands of times more massive. The solar wind is forming swirling eddies and vortices in its wake as it carries magnetic fields with it. The corona has such a high temperature that the Sun's gravity is letting the solar wind escape. The solar wind is composed of ionized particles which are moving at speeds of between 250-500 miles per second (400-800 km/s). The solar wind is mostly made of hydrogen. It's measured by its speed and by its proton density. With a speed ranging 0.9-1.8 million mph (1.5-2.9 million km/h) the solar wind is reaching Earth in 4 to 8 days. The solar wind displays a complex and dynamic flow. With high speed solar winds course off the Sun, overtaking take slower winds, and creating shock waves to form giant, spinning eddies known as co-rotating interaction regions (CIRs) and these CIRs are an important component in accelerating particles. Such a flow is the most usual product of the Sun. It's the usual entrance of the solar wind into the Earth's magnetosphere which is producing the auroras. It seems now well established that the solar storms and the dangerous radiation are yielded by fast CMEs (see below) which are plowing into a slow solar wind ahead of them. This builds a shock front which is accelerating the solar wind particles. A crude way to estimate the speed at which the solar wind is leaving the Sun was, until now, to ask whether it was leaving the Sun from a zone where the magnetic loops were closed, or from a zone where they were opened (like in the coronal holes found in permanence at the poles, or sometimes elsewhere at the surface), bringing to a slow -but dense, or a fast -but thin- solar wind respectively. A study in 2005 is showing that characterizing the chromosphere under from where the solar wind is originating allows to predict both the speed and the isotopic composition of it, providing a better way to predict solar storms and radiation. The chromosphere has been found shallow, dense, and compressed beneath area of closed magnetic loops, as it was found thicker and less dense under the coronal holes. In this later case, the thicker the chromospheric layer is, the more it is being allowed to expand by open magnetic fields and the faster the solar wind will blow. This new method is more precise than the old 'fast or slow' estimate. Such coronal holes appear like dark regions in the SOHO EIT 195 pictures. The fast solar wind, on the other hand, has been further accurately located in a source region lying between 3,100 and 12,400 mi (5,000-20,000 km) above the surface. The closed magnetic loops there are swept by convection to funnel regions where they reconnect with existing open lines. This reconnection allows the plasma trapped into the closed lines to escape, and to be accelerated! The acceleration of the solar wind appeaers also to be powered by a special kind of waves that can accelerate certain particles preferentially. Finest detail of the solar wind seen when a study zoomed in it close to Earth, found that tiny turbulent swirls, or turbulence, could play a big role in maintaining its heats, and counterbalancing the natural tendency of the solar wind to cool down. Turbulence arises from irregularities in the flow of particles and magnetic field lines, but understanding how this energy is transferred from the large scales where it originates, to the small scales where it is dissipated is difficult. That shows how the solar wind plasma is extremely structured at a high resolution. Astronomers already had seen that current sheets on scales of 62 miles (100 km) in the magnetosheath are turbulent eddies too and that, at their border, magnetic reconnection events have been spotted as oppositely directed field lines spontaneously break and reconnect with other nearby field lines, thus releasing their energy. It is unknown whether reconnection is occurring as smaller scales albeit new observed eddies are at the end of a cascade of energy which, anyway, is heating the solar wind. It is unknown too whether such small scale eddies occur closer to the Sun
->More for the Solar Wind from The Margins of the Active Regions!
As the solar wind usually considered forming in the solar active regions (the regions of the solar sunspots), it might that those flux be perturbed -and accelerated up to speeds of 150,000 mph (240,000 km/h), with temperatures between 1 and 3 millions degrees Celsius- from a still badly known activity at the margins of those regions. Such a contribution might be an important one to the total volume of the solar wind, with the equivalent of billions tons a day by an active region!
- The Interplanetary Magnetic Field (IMF). The Interplanetary Magnetic Field (IMF) is the other usual component of the solar flow. Most of the time, the Sun's magnetic field is similar to the one of the Earth. It's magnet-shaped, that is a dipole with a North-South polarity. Great closed loops exist at the equator's level as opened lines pour from the poles. The IMF is interlaced with the solar wind. Due to the fact that the Sun is rotating in 27 days, the IMF is spiral-shaped (this spiral is called the 'Parker spiral'). The Sun’s magnetic field, which is embedded in the solar wind, permeates the
entire solar system. The 'heliospheric current sheet' is where the magnetic field changes
polarity and spirals out from near the solar equator. The IMF is interacting too with the Earth's magnetosphere. When it's oriented South, that is at the opposite of the Earth's magnetosphere, the two fields are reconnecting together, which yields a crack in the magnetosphere. This is what is called the phenomenon of the 'reconnection'.
Although the study of magnetic reconnection occurring on the Sun, in Earth's magnetosphere or in nuclear laboratories, dates back to the 1950s and despite
numerous scientific papers addressing this perplexing issue, scientists still
cannot agree on one accepted model. Such a puncture may occur at 38,000 mi (61,000 km) above the Earth, as it's as large as twice the Earth. The solar wind is entering these cracks down to the upper atmosphere, where it produces auroras. Recent findings showed that the cracks may last up to 9 hours as the auroras they produce are visible more South than usual due to the unusual solar magnetic field's strength
- Strahls. Another kind of particle flow are jets of high-energy electrons streaming from the Sun known as 'electron strahl.' That are no extra-normal events but the fact that solar fast-moving electrons are travelling along the Sun's magnetic field lines that flow out from the Sun, some looping back to the Sun, other extending out to the edge of the solar system. The charge on an electron interacts with the field lines such that each
particle sticks close to the line and gyrates in circles around the field lines at the same time. As the magnetic fileds get weaker further from the Sun, a physical law demands that, so electrons are not pushed off course from the line, the gyrations get smaller and more stretched
out along the field line. The strahl phenomenon thus should become a more and more focused which has not been found at Earth, with a strahl larger than thought. Strahls along open field lines, those that do not return to the Sun, have different characteristics than those on closed field lines, those that do return to the Sun as strahls on closed lines may have varied width, generally. The number of electrons passing by in all cases however is remaining the same. Electromagnetic waves might be the key factor to strahl. Themselves, on a other hand, are numerous in types traveling at different speeds,
in different sizes and in different directions, and no one yet knows which kinds
of waves might be at work
- Coronal Holes. Coronal holes are low-density regions of the Sun’s atmosphere, known as the corona. Because they contain little solar material, they have lower temperatures and thus appear much darker than their surroundings and visible in certain types of extreme ultraviolet light. While it’s unclear what causes coronal holes, they correlate to areas on the Sun where magnetic fields soar up and away, without looping back down to the surface, as they do elsewhere on the solar surface. Coronal holes are dark -and relatively cool in temperature- areas in the solar corona as seen in the ultraviolet. Coronal holes appear as dark areas in extreme ultraviolet light,
because there is less material in the hole to give off light in these
wavelengths. Coronal holes are large regions in the solar corona that appear darker and are less dense and cooler than surrounding areas. The open structure of their magnetic field into space allows a constant flow of high-density plasma to stream out of the holes. The high-speed solar wind is known to originate in coronal holes, specially out of their center, at speeds up to 500 miles/s as wind flowing out of the sides slows down as it expands into space. These holes are often sources of solar wind gusts which may take away solar particles into space. Such solar wind streams then reach Earth where they may yield geomagnetic storms. Coronal holes are zones of weak solar magnetic field. This allows high speed solar wind particles to escape. These gusts take 2-3 days to reach Earth. Solar wind escaping from large coronal holes are of high speed. Usually lines of the Sun's magnetic field are falling back to the Sun's surface, but, as far as coronal holes are concerned, lines stretch into the solar wind. A pattern of cells
with bright centers and dark boundaries have been seen occurring in the
corona. Coronal cells occur in areas between coronal holes and solar filaments which mark the boundaries between sections of upward-pointing magnetic fields
and downward-pointing ones. They might affect the steady emission of the solar wind
streaming from these holes. As seen from the side such cells look like a candle flame and like a cell as seen from the sky and likely are columns of solar material extending upwards like giant pillars of gas. The complex solar magnetic fields have also been seen laying centered inside the cells which makes a clear distinction between the coronal cells and supergranules. Supergranules also appear as a large
cell-like pattern on the Sun's surface, and their delineated edges are created
as the sideways motion of solar material sweeps weaker magnetic fields toward
their boundaries. Supergranules, therefore, appear to have enhanced magnetic
fields at their edges, while the coronal cells show them at their centers. The cells consistently occurred in areas dominated by
magnetic fields that point in a single direction, either up or down. In
addition, the fields of the nearby coronal hole are what's known as 'open' ones
extending far into space without returning to the Sun. On the other hand, the
field lines in the cells were 'closed,' looping up over the filament and
connecting back down to the Sun. A region of coronal cells may turn into a coronal hole when a filament erupts from the adjacent area. Jets occurring from coronal holes are explained by the Emerging-Flux Model, as a new flux coming up to the photosphere and its interaction with the
photosphere’s complex magnetic topology creates the jets. Jets were eventually found being caused by scaled down filament eruptions. Coronal holes are usually located near Sun's poles but they may occur elsewhere too. A periodicity of about nine days when the solar radiation linked to a coronal hole is reaching Earth is observed, due to that coronal holes, which are at the origin of the strong solar winds, are positioned 120 degrees apart on the sun’s surface (as the sun rotates every 27 days, these solar winds typically hit Earth every nine days!). The periodicity may turn into a seven-day one, indicating that a fourth hole opened up. Such periodicities seems to have appear only during the last solar cycle. Coronal holes are visually assessed and whether they are or not linked to a solar wind stream reaching Earth as a G scale allows to assess the strength of ensuing geomagnetic storms, a R scale the radio blackouts and a S one storm radiation
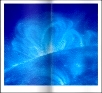 | UV view of magnetic loops above sunspots as seen at the solar limb. picture SOHO |
- Solar Sunspots. Solar sunspots are places of intense and complex magnetic loops in the solar magnetic field, which are partly controlling the outflow of the solar plasma. Magnetic fields can restrict the plasma's flow and create spots as changes to the Sun's magnetic field can thus affect the number and distribution of sunspots. Also termed 'active regions,' they are areas of very concentrated magnetic field. Charged solar particles travel along and illuminate magnetic field lines above solar active regions. Charged particles spiraling along these magnetic fields emit extreme ultraviolet light, helping to trace out the magnetic field lines. The temperature is so hot in the Sun's outer atmosphere that atoms are colliding there stripping each other from their electrons. Hence the atoms part into positively charged ions on one side and negatively charged electrons on the other. This is known as a plasma. This on the other hand is trapping this material along the lines of Sun's magnetic field. Spots may also be viewed like a consequence of rearrangement and realignment of Sun's magnetic fields. As the solar plasma is mostly the steady outflow of the solar wind, sunspots are at the origin of more unusual events. A 'delta region' in which the lighter areas around the sunspot, the penumbra, exhibit magnetic fields that point in the opposite direction of those fields in the center brings a fairly unstable configuration that can lead to solar flares. When the pressure of the solar material generally, builds up, the magnetic lines eventually break, leading either to a solar flare when the burst occurs near the surface, or to a Coronal Mass Ejection (CME) when it occurs in the solar corona. The magnetic field of the sunspots is hundred times stronger than the usual solar magnetic field. Hence they add to the latter making the magnetic field at the surface of the Sun tangled and complicated. Magnetic loops seen above the sunspots are tubes of magnetic flux where a plasma of ions and electrons are magnetically trapped. Due to heat, atoms are colliding, stripping off their electrons. At a sunspot region, the magnetic field lines, at the edges of each sunspot, bend over to reconnect with field of opposite polarity. Sunspots are often seen accompanied by bright areas called 'faculae,' which are regions of magnetic activity too and mostly the mark that there was once a sunspot group there. A significant amount of soft X-rays occurs at the Sun at even a small amount of magnetically complex sunspots as they might well be a result of nanoflares, or they might have their origin from flares. Sun's soft X-rays, generally, constitute a particular type of light from the Sun as that light is highly variable and can impact Earth’s upper atmosphere. Sunspots are estimated by the sunspot number (see the tutorial 'Observing the Sun') and are assessed as being or not sources of solar flares. Sunspot areas, or 'active regions,' have strong magnetic fields. These are areas of intense and complex magnetic activity that can sometimes give rise to solar eruptions such as solar flares and coronal mass ejections. Twisted magnetic fields within the active region trap the hot, charged particles on the Sun, called plasma making them hotter and often denser than surrounding areas. As the magnetic fields twist, they stretch and stress until they snap and reconnect into a simpler configuration. How and when a particular active region is to erupt is still a outstanding science question. When magnetically active regions are close-set, magnetic field lines create a tangle of arches snaking through the solar atmosphere with charged particles spinning along them, emitting extreme ultraviolet light. Magnetic field lines are constantly connecting, breaking apart, and reconnecting among the several active regions
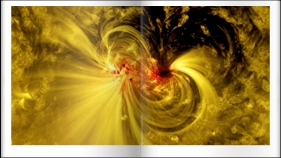 | A blended view of a sunspot in visible and extreme ultraviolet light, revealing bright coils arcing over the active region, or particles spiraling along magnetic field lines. picture NASA’s Goddard Space Flight Center/SDO |
->Flares and CMEs
A flare is a high-power energy release per unit time, a mostly radiative energy release event , and the most powerful storm in our solar system, as A CME is a lower power event occurring over a much longer time period, although carrying lots of mechanical (kinetic) and magnetic (potential) energy. CMEs blast out over a billion of tons of particles at millions of miles per hour. Solar electrons and ions accelerated up to near the speed of light affect all Sun’s atmospheric layers and pass through the corona, which may add some also. Post coronal loops can be seen coiled over the area where a flare occurred, lingering during about one Earth's day. Sometimes the process which leads to a flare and a CME is contradicted when one mass of magnetized material, instead of colliding with another one, just encounter a invisible boundary called a 'hyperbolic flux tube.' A hyperbolic flux tube is the result of a collision of two bipolar regions, a magnetic complex structure of four alternating and opposing magnetic fields ripe for magnetic reconnection. The tube may, for example, reconnect a filament’s magnetic field lines with those of the ambient Sun. Regular pulses disturbances -- akin to earthquakes -- are rippling through the chromosphere during a flare as they are later observed like oscillations in the corona
- Solar Flares. A solar flare is an intense burst of radiation coming from the release of
magnetic energy associated with sunspots. Solar flares are the result of a magnetic reconnection, which was not recognized before 1949 as the flares had been known since 1859. Solar flares are giant explosions on the Sun that send energy, light and high speed particles into space. During flares, a large amount of magnetic energy is released, heating the Sun’s atmosphere and releasing energized particles out into space. Post-flare loops or coronal rain then are falling back to the solar surface. Flares are our solar system’s largest explosive events. They are seen as bright areas on the Sun and they can last from minutes to hours. The beginning of flares is when the interesting physics happens. A solar flare is typically observed by the photons (or light) it releases,
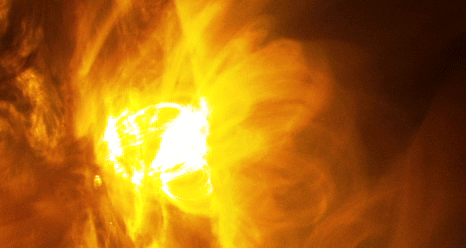 | Giant magnetic loops work in concert with the eruption of a solar flare, seen as a bright flash of light. picture NASA/SDO |
at most every wavelength of the spectrum. Flares are also sites where
particles (electrons, protons, and heavier particles) are accelerated. Solar flares are explosions happening when the energy stored in magnetic fields looping above sunspots in loops tens of times the size of Earth is suddenly released. A flare may be released like a huge energy between two spots, one higher in the Sun's atmosphere and the other one lower. 'Sympathetic' flaring is often seen on the sun, where an outburst in one active region triggers a blast
in another. During a solar flare, the gas soars to over 20 million degrees Fahrenheit, and emits X-rays that scientists can use as fingerprints to study these events on the Sun. During a flare, X-rays generally represent
electron activity as gamma rays activity from protons and other heavier charged particles called ions. It is likely that different circumstances guide the movements of different particle populations with perhaps the ions accelerated in a different way and end up traveling on different magnetic field lines than the electrons. A flare is a quick, large scale change in the solar magnetic field, and a radiation event. The existence of a current sheet is crucial in all models of solar flares. Current sheets form when two oppositely-aligned magnetic fields come in close
contact, creating very high magnetic pressure. Electric current flowing through this high-pressure area is squeezed, compressing it down to a very fast and thin sheet. This configuration of magnetic fields is unstable, meaning
that the same conditions that create current sheets are also ripe for magnetic reconnection. The explosion occurs at the top of the loop, as it heats the solar atmosphere and accelerates electrons, which runs down each leg of the broken loop! Release expels radiations ranging from radio waves to X- and gamma-rays. X-rays are part of solar flares events in terms of they can disturb near-Earth space. A late study through NASA's RHESSI showed that solar flares are a reconnection of magnetic field when the latter is stretched away from the Sun under the pressure of ejected solar plasma. Reconnection heats the Sun's atmosphere to tens of millions of degrees as the solar plasma is accelerated to almost the speed of light. It's why solar flares are shining in the X-rays. It was found lately that emerging magnetic fields from the solar interior which are not in alignment with the pre-existing field at some place, and interacting with it, are generating strong electrical current which, building on several hours, leads with a strong probability to a flaring activity. Solar flares were thought as potential culprits for the heat of the solar corona, but a 2003 study showed that microflares (a million times smaller than flares and far more frequent) might as a whole account for that. Microflares are much smaller energy releases and they all occur
in active regions over the same range of latitudes on the Sun as for the bigger events, so they maybe are just smaller versions of the same phenomenon. Microflares rapidly release energy and heat the material in the active
regions. Important flares, on the other hand, have their powerful explosions yielding influence unto the slow oscillations which usually occur and exist in the convective, outermost part of the Sun's interior. Solar flares are sorted according to their brightness in the X-rays range and to their strength. There are 3 categories (X, M, C), each with a 10-fold increase, and they are subdivided into nine finer subdivisions from 1 to 9; there is too a A (background level) and B category. X class flares are major events which may yield radio blackouts for the entire Earth and long duration, intense radiation storms. The most powerful flare measured with modern methods was in 2003, during the last solar maximum, and it was so powerful that it overloaded the sensors measuring it. The sensors cut out at X15, but the flare was estimated to be as high as an X28. Radio effects from a flare are felt at Earth 8 minute only after the explosion at the Sun, which the time the waves travel at light speed. Class M flares are medium events yielding short duration radio blackouts mainly in polar regions as C class flares are minor events without noteworthy consequences for Earth. Solar flares may be very harmful, like the solar storm in 1859, one of the largest on record, caused telegraph systems do fail worldwide. Most powerful flares generate too bright auroras. A pecular form of flares have been observed in 2008, showing that those ones are getting hotter as they just focus on heating the solar atmosphere instead of dividing their energy between that and accelerating particles, thus becoming 9 million of degrees hotter. The white light flares are constituting a large percentage of the occurring flares and are the result of a associated brightening in the visible light spectrum. As they are unfrequent, the mechanism for their creation remains unclear as highly accelerated electrons to 40 percent of the light speed might be responsible, as correlated with hard X-ray emissions and linked to sunspots. As they originate at the solar surface thus, white light flares are bound to 620 miles above, into the chromosphere. Modeling energy transfer of high-energy particles in the solar
atmosphere is an important subject for further improving human understanding of
particle acceleration. At the occasion of a flare the Sun can also emit streams of very fast protons – known
as solar energetic particle (SEP) events – and disturbances in the solar wind
known as corotating interaction regions (CIRs) and both can also produce a
variety of solar storms on Earth. The most intense consequences of a flare event -even a weak one- can cause a shower of particles to cascade down toward Earth's surface as that shower is called a ground level enhancement (GLE). GLEs are quite rare -fewer than 100 events have been observed in the last 70
years. Wave-particle interaction known like Whistler waves, a phenomenon seen with CMEs at the Sun, might also happen in supernova blast waves. 'Superflares' -enormous explosions- were frequent when the Sun was a young star, now are so rare that they are experienced each 100 years or so only
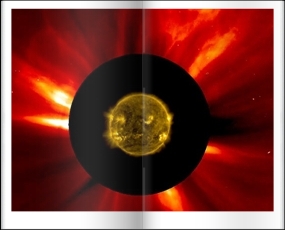 | Sun's innermost atmosphere as
seen by the Solar Dynamics Observatory (SDO) inside a larger image provided by
the Solar and Heliospheric Observatory (SOHO) is showing how there may be continuity, or not, between solar events like between bright, yellow area in the inner view and bright area in the outside one. The coronal mass ejection or CME traveling away from the Sun in the upper right corner however does not correlate with any event in the inner view. picture ESA/NASA/SOHO/SDO/GSFC |
- Coronal Mass Ejections (CME). A CME is a vast cloud of electrically charged particles, with a magnetic field, hurled into space. The nascent shock of a CME is crescent shapes and when it expands, it turns ellipsoid as a CME features a strong shock near its nose and a weaker one at the side. When a CME with its magnetic disturbances, encounters the Earth's magnetic field, they interact in a series of complex physical processes, with those perturbations called geomagnetic storms. Where the strong magnetic fields found in the solar corona are extant and closed, often above sunspot groups, the confined solar atmosphere can suddenly and violently release bubbles of gas and magnetic fields
called coronal mass ejections, or CMEs. CMEs are huge, fast-moving clouds of electrically-charged solar material that contain embedded magnetic fields, can cause geomagnetic storms when they collide with Earth’s magnetic field, causing it to shimmy and shake. CMEs are sometimes associated with flares but they can occur independently. A solar flare followed by a CME may further be accompanied with a phenomenon known as 'coronal rain!' It is when hot plasma in the corona cools and condenses along strong magnetic fields in the region. Two broad theories exist about how the magnetic energy of a CME develops. First, a situation in
which a series of loops of magnetic fields known as an 'arcade', is the start of every active region CME. This arcade has a weak point at the top, a place where the energy from below can burst through once it's great enough. During the eruption a flux rope forms. The second model assumes that the flux rope is there before the CME erupts. In this theory, no weak point is required. Instead, the flux rope gains more and
more energy, and becomes increasingly unstable until a disturbance on the Sun causes it to release the energy out into space. It is that second model which later studies proved true and the ability to unleash a CME foreseeable from a sunspots' magnetic region under, at a critical value. There is also a critical height for rising flux rope, beyond which the magnetic loops above can no longer keep it confined. CMEs generally are large gas bubbles mixed with lines of magnetic field and expelled from the Sun on a duration of several hours. CMEs take place when Sun's magnetic field lines over the sunspots are stretched up into the solar corona, and that an increasing pressure leads them to a point that they snap. Billion tons of solar plasma are ejected in the solar system, travelling at speed over a million mph and carrying away some of the Sun's magnetic field with them! CME speeds with distance from the Sun is not well understood, in particular in the outer solar system. Complex and distorted magnetic fields, bearing ionized particles, are travelling along with a CME, perturbating the solar wind flow. A large, moving disturbance is thus occurring in the solar system. CMEs are eventually reaching Earth after a journey of two to four days (fastest do in about 40 hours), disturbing the Earth's magnetosphere and upper atmosphere, triggering an intense activity. Magnetic fields sweeping around the Sun are crucial to understanding how CMEs travel through space. The magnetics fields may be considered the 'skeleton of the entire heliosphere,' that blob encompassing the whole solar system. They thus are guiding how particles and CMEs move toward Earth as astronomers routinely measure the fields near the Sun's surface. Measurements in the upper corona needs are also possible by studying the bow shocks created by a CME function of the 'Alfvén speed' which governs how fast waves can propagate
through plasma and determining the strength of the magnetic field. CMEs are carrying a magnetic field, with a polarity, away from Sun. Like what occurs with the Interplanetary Magnetic Field, when the CME's magnetic field is South, it's interacting with the Earth's magnetosphere. When North the Earth is shielded by its own field. CMEs polarity may not be determined however before they reach monitoring satellites in orbit, that is about 15 minutes before reaching Earth. Such an activity may harm satellites and power grids, and threaten astronauts with high radiation doses. CMEs are increasing the aurora activity too, bringing auroras much more South (or North) than usual. When a southward solar magnetic field interacts strongly with the oppositely oriented magnetic field of the Earth, the Earth's magnetic field is then peeled open like an onion allowing energetic solar wind particles to stream down the field lines to hit the atmosphere over the poles. At the Earth's surface a magnetic storm is seen as a rapid drop in the Earth's magnetic field strength. This decrease lasts about 6 to 12 hours, after which the magnetic field gradually recovers over a
period of several days. When a CME is reaching Earth, its size is about 30 million miles (48 million km), that is about the orbit of Mercury! At the moment of the cycle maximum, there are 2-3 CMEs a day; at the moment of the cycle minimum, there are 1 CME a week. Some CMEs are this important and directed to Earth that, in perspective, they form an halo around the Sun (they are called 'halos CMEs'). Solar tsunamis often happen in close conjunction with CMEs, giving scientists the first clues as to whether halo CMEs were heading directly toward or away from Earth. CMEs are often associated with solar flares and large prominences (these filaments are huge clouds of relatively cool dense plasma, suspended in Sun's corona by the solar magnetic field, the same complex magnetism that drives solar events like flares and coronal mass ejections. The solar material in the prominence streams along the sun’s magnetic field lines before it thins
out and gradually breaks away from the solar surface) but they may exist independently too. A filament indeed is a structure in the corona consisting of cool plasma supported by magnetic fields. Filaments can be very large, more than 68,000 miles long as they are often unstable and usually break apart in less than a week, though they can last longer than that. Filaments are usually elongated and uneven strands as only a handful of were observed like a circle. They stem from the photosphere and keep suspended in the corona. Filaments can end their life under the form of cascading magnetics arches triggerring all along. Changes in magnetic fields around filaments can cause them to erupt and result in solar flares and coronal mass ejections (CMEs). Above proeminences, coronal jets -- which are often observed in the polar regions of the Sun -- are opened magnetic structures which may reach beyond 10 solar radius before to vanish. An accompanying phenomenon to a solar flare-CME event is a proton storm. Solar protons are accelerated to nearly the speed of light by the explosion and they are reaching Earth minutes only after the flare, or they may take longer, about two hours or more. This is what is called a 'proton storm'. It may last some days as such highly electrified protons can penetrate 4.3" of water and are delivering radiation doses to any astronauts above any protective planetary magnetosphere. Scientists are still debating whether the energetic particles from such events are accelerated by the flare itself, by the shock front of an associated coronal mass ejection, or both. Magnetic fields inside a CME are spiral-shaped and are traceable through the CME gas (a plasma), which is showing this shape. A 2004 study using SOHO LASCO images managed to visualize such a CME travelling in space in three dimensions. A CME is an expanding swarm of arcades of loops, rather than a bubble or rope-like structure. Loops are remaining connected to the source region longer than thought, at least as long as the CME is visible inside SOHO LASCO field. A study at the Solar Physics Division of the AAA, June 2003 Annual meeting (Laurel, Md) showed that CMEs work like solar flares. Stretched magnetic fields snap and re-combine releasing a strong energy, and a 2003 study showed that fastest and largest CMEs are really linked to solar flares -CMEs have their source into the burst of a flare, as smallest CMEs would arise from another kind of magnetic field, which do not snap -because not compressed enough, but only gently rise, rising the CME with it into the corona. CMEs occur when there is a quick and large scale change of the Sun's magnetic field. The cannibal CMEs, a sort of CMEs, may be the source of 'complex ejecta' CME clouds, with a
larger and more complex structure than typical CMEs, triggering protracted magnetic storms when they are reaching and enveloping Earth. CME may carry away up to 10 billion tons of plasma to speeds reaching 3,200 miles per second (2,000 km per second) -millions of mph (or km/h); with records at 4650 miles/s -2,890 km per second). The geomagnetic storms they are generating at Earth are categorized into active, minor and severe. The CMEs are interfering with communications between ground stations and satellites, airplane pilots, and astronauts. Radio noise from a storm can also disrupt cell phone service. Disturbances in the ionosphere caused by CMEs can distort the accuracy of Global Positioning System (GPS) navigation and, in extreme cases, induce stray electrical currents in long cables and power transformers on the ground. Electromagnetic pulses from geomagnetic or solar storms are now considered a potential danger to the US electric grid that they are part of the threats considered by the The Grid Reliability and Infrastructure Defense Act (H.R. 5026) due to modern societies having technologies with a sensitivity to solar storms. New viewpoints think that such threats might be larger than previously thought, up to widespread tripping of transmission lines or irreversible damage to large transformers. EMP, which are high-altitude pulse caused by a solar
event are specially feared bringing between $1
trillion and $2 trillion in damages and 10 years to recover. Latest researches are showing that flares and related CMEs are triggered from the solar atmosphere, and not surface like previously thought, much more like lightning, with energy slowly accumulated and suddenly released. Two theories until now were explaining why CMEs start with a gigantic arch, or coronal loop with both feet planted into the photosphere (coronal loops usually are found around sunspots and in active
regions and associated with the closed magnetic field lines
that connect magnetic regions on the solar surface. Coronal loops are the solar coronna's building blocks. Many coronal loops last for
days or weeks, but most change quite rapidly. It looks like the apparition of a arch does not surge from the surface but from energy which oozed up slowly with time and storing up in the atmosphere until released in a
massive explosion of light, plasma, magnetic fields and high energy particles, and a coronal loop. The results however have
created more mystery as the question whence the energy triggering the release comes from remains unsolved: material moving perpendicular to the
magnetic field at the base of a coronal loop, or 'crux,' for example, is moving too slowly, at speeds 10,000 times less than is expected if it were
directly triggering the eruption. CMEs occur in the solar corona. Prominences, at the origin of CMEs, are sometimes also the inner structure of a larger formation,
appearing from the side almost as the filament inside a large light bulb. The
bright structure around and above that light bulb is called a streamer, and the
inside area is called a coronal prominence cavity. A giant inner tube of looping magnetic fields also comes with the coronal cavity as temperatures vary greatly throughout (hotter and cooler areas link the much colder 17,000 F (10,000 degrees Celsius) prominence at the
bottom to the 1.8 million to 3.6 million
degrees Fahrenheit (million to two million degrees Celsius) corona at the top). A cavity is less dense by 30 percent than the streamer which remains high in a sense (such hollows only look empty compared to their surroundings). Magnetic fields likely are creating, and supporting such cavities which likely look like a
tunnel in a crescent shape, not unlike a hollow croissant. Cavity features are constantly in motion creating a complicated flow pattern as, sometimes, these cavities can be stable for days and
weeks, but then suddenly erupt into a CME. The US weather agency, the NOAA, measures geomagnetic storms on a five-point scale from 1 to 5. G1 storms
are minor, leading to weak power grid fluctuations and having only minor impact
on satellites. G5 storms are extreme, leading to widespread voltage control
problems, damage to transformers, radio outages and satellite problems. CMEs move so much
faster than the background solar wind that they create shock waves in it with the wave picking up particles that are traveling more slowly and speeds
them up, piling them up in front as it moves. Massive Whistler waves are waves propagating obliquely to the magnetic field might be created by instabilities out in front of the shock as they both increase particles' bulk
kinetic, and random energy (which relates to the speeds at which particles move
with respect to each other and increase their temperature). They might increase the random
kinetic energy of the positively-charged ions in the solar plasma in a direction perpendicular to
the background magnetic field and also add bulk acceleration of electrons in a direction
parallel to the latter. When CMEs are reaching Earth, they feature a typical balloon shape. Beyond Earth, because particles
must travel for many months before reaching the outermost reaches of the solar system, the CMEs eventually spread
out and merge with other CMEs and the solar wind to form larger clouds of
particles and magnetic field. These combined clouds stretch out as they travel
away from the Sun, forming thin ring shapes by the time they reach the boundaries of Pluto's orbit. Compared to typical CMEs, 'stealth CMEs' are moving at between 250 to 435 miles per second only, or roughly the speed of the solar wind. They can cause minor to moderate disturbances to Earth’s magnetic field as they might originate from the Sun’s differential rotation which may entangle magnetic lines
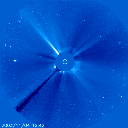 | 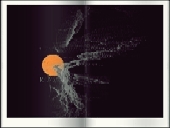 | Here is an example of a series of CMEs as seen by the SOHO spacecraft in November 2003 as solar activity was particularly active. CMEs here illustrated unfolded on a real timespan of 11½ hours. First of them is probably an halo CME. SOHO is using a coronograph to block Sun's light; central white circle is Sun's real size. Right is seen a 3-D modelized CME following a new technique developped in 2004 (click for a larger picture). pictures courtes3 Soho observing the Sun (left) and NASA's Goddard Space Flight Center (right) |
- Interplanetary Shock Waves. Interplanetary shockwaves are caused by halo CMEs. They are due to the fact that materials which are expelled from the Sun at high speed hit previouly ejected, and slower matter. Hence energy is stacking and increasing in density. The solar wind becomes denser. Interplanetary shock waves are carrying an associated magnetic field which has a North or a South component. When the component is South (i.e. opposite to Earth's magnetic field), shock waves may cancel magnetosphere at the point where they reach allowing the solar wind to pervade into the magnetosphere. This often triggers auroras. Another more dangerous consequence of a shock wave is that the CME is accelerating protons, producing a proton storm at Earth. Such high intensity protons may be harmful to spacecraft and astronauts
Website Manager: G. Guichard, site 'Amateur Astronomy,' http://stars5.6te.net. Page Editor: G. Guichard. last edited: 4/15/2019. contact us at ggwebsites@outlook.com